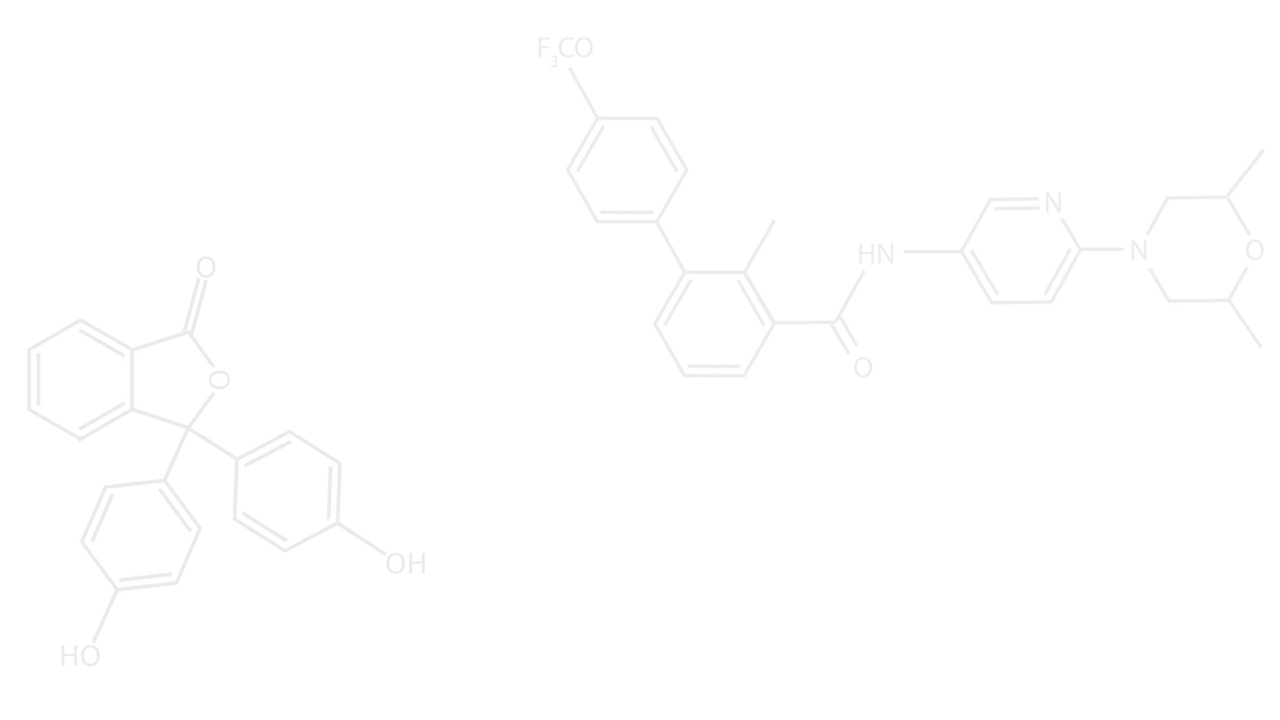
RESEARCH
Two-dimensional acoustic black holes for flexural waves
Circular indentations of power-law profile act like two-dimensional acoustic black holes for flexural waves in plates. This means that a substantial part of energy of incident flexural waves is absorbed by them, which can be used for efficient damping of structural vibrations in plate-like structures. The actual acoustic black hole pictured below should also have a small piece of absorbing material (e.g. rubber) attached to the centre (not shown on the photo) to provide energy absorption.
The advantage of using acoustic black holes over traditional methods of vibration damping is that they require very small amounts of absorbing materials. Their disadvantage is in the requirement to drill the holes in plates, which limits their applications only to specific types of practical constructions that tolerate the presence of holes. A more detailed information can be found in the paper by Krylov, VV and Bowyer, EP (2013), Acoustic Black Holes: A New Approach to Vibration Damping in Light-weight Structures, Proceedings of the Institute of Acoustics, 35(1), pp.184-191.

View of a two-dimensional acoustic black hole manufactured in a rectangular steel plate.
Wedge elastic waves: applications in non-destructive testing and marine engineering
Wedge elastic waves are localised waves propagating along sharp edges of elastic wedges (see figure). There is a finite number of modes of wedge waves propagating at different velocities. If a wedge is formed by two intersecting planes (a linear wedge), the velocities of all modes of propagating waves are non-dispersive (do not depend on frequency). If a wedge has a more general form, for example a truncated linear wedge, all modes become dispersive, i.e. their velocities are functions of frequency. Wedge elastic waves have been first predicted in 1972 using numerical calculations (Lagasse, 1972; Maradudin et al, 1972). Later on, their existence has been confirmed experimentally. Current interest in wedge elastic waves is partly motivated by their potential applications in ultrasonic non-destructive testing (for examining structural integrity of specific wedge-like structures - with sharp edges) and in marine engineering (for providing wave-like aquatic propulsion of marine vessels, similar to that used by some marine animals, such as stingrays). Further details can be found in:
[1] Krylov, VV (2016), Wedge Elastic Waves, with Applications to Ultrasonic Non-destructive Testing. In 55th Annual British Conference on Non-Destructive Testing, 'NDT 2016', Nottingham, UK. Full text: http://www.bindt.org/events/NDT-2016/?afc=PromoteIT_55682 .
[2] Krylov, VV and Porteous, E (2010), Wave-Like Aquatic Propulsion of Mono-Hull Marine Vessels, Ocean Engineering, 37, pp. 378-386, ISSN: 0029-8018. Full text: http://www.elsevier.com/locate/oceaneng. DOI: 10.1016/j.oceaneng.2009.12.008.

Wedge elastic propagating along the tip of a slender wedge.
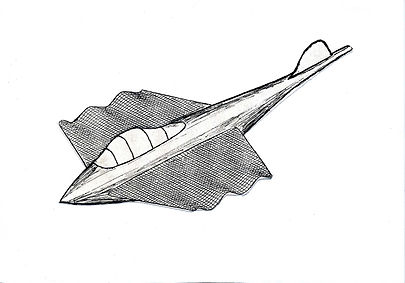
A small submarine propelled by propagating wedge elastic waves (Krylov, 1994).
On the possibility of ground vibration boom on the proposed HS2 high-speed railway lines
One of the most serious engineering and environmental problems associated with high speed trains is a dramatic increase in the level of railway-generated ground vibrations that can occur when the train speed exceeds the velocity of Rayleigh surface waves in the supporting ground. This phenomenon has been first predicted theoretically more than 20 years ago (Krylov, 1994a), and it is currently known as 'ground vibration boom', because it is similar to the well-known phenomenon of 'sonic boom' from supersonic aircraft that occurs when the speed of aircraft exceeds the velocity of sound in air (see Figure).
The essential difference between sonic boom and ground vibration boom is in the fact that, whereas sound velocity in air is roughly the same in all locations above the earth surface, the Rayleigh wave velocity in the ground is different in different locations, depending on local geological properties of the ground. In some locations, where the ground is soft and marshy, the Rayleigh wave velocity can be very low, so that it can be exceeded by high train speeds. And it is such 'sensitive' locations that may represent the risks of occurrence of ground vibration boom from operating high-speed trains. Some preliminary estimates show that the proposed HS2 high-speed routes connecting London with Northern England via Birmingham at speeds up to 400 km/h may give a possibility of ground vibration boom to occur in some sensitive locations.
As a rule, train speeds even as high as 400 km/h are still lover than Rayleigh wave velocities in the ground in the majority of locations. However, in sensitive locations characterised by low Rayleigh wave velocities the phenomenon of ground vibration boom will represent a serious environmental hazard to be reckoned with. Further details can be found in the paper by Krylov, V. V. and Lewis, B. (2016), Assessment of Locations Along the Proposed HS2 Routes that are Likely to Experience Ground Vibration Boom from High-speed Trains, Proceedings of the Institute of Acoustics, 38(1), 453-464.
​

Rayleigh surface waves generated by high-speed trains at ground vibration boom; waves are generated at Mach angles.
Reduced-scale modelling of structure-borne vehicle interior noise
Although studies of structure-borne vehicle interior noise based on detailed numerical calculations have achieved satisfactory levels of accuracy in predicting interior structural-acoustic response, they are not much helpful in understanding physical mechanisms behind the problem. A better understanding of such mechanisms would be of great importance for predicting the behaviour of new vehicles on a design stage. Therefore, it is important to develop new approaches to studying vehicle interior noise based on applying simplified reduced-scale vehicle models. Such models can be easily used for different laboratory measurements, and, due to their simplicity, they can assist in better understanding the physics of structure-borne interior noise, in particular its dependence on different parameters of vehicle structures and interior cavities.
One of the promising ways to use reduced-scale models is by applying the combined finite element and experimental approach to studying structure-borne vehicle interior noise. In the framework of this approach, new reduced-scale vehicle models of intermediate complexity have been designed and tested both numerically and experimentally. A number of finite element calculations of structure-borne vehicle interior noise have been carried out. Experimental tests included measurements of structural-acoustic frequency response functions at driver’s and passenger’s ear positions, when an electromagnetic shaker was located at different parts of the vehicle model structure, thus simulating different sources of vehicle structural vibrations. In addition to these, the effects of engine mass and of boot load on generated structure-borne interior noise have been investigated. The results of some of the finite element calculations have been compared with the relevant experimental measurements. Fore more detail, see the paper by Georgiev, V. B., Ranavaya, R. L. and Krylov, V. V. (2015), Finite Element and Experimental Modelling of Structure-borne Vehicle Interior Noise, Noise Theory and Practice, 1(2), 10-26.

Photo: Simplified reduced-scale vehicle models used for investigations of structure-borne interior noise.